Nitrous oxide- discovered by Joseph Priestley in 1772 and known affectionately as “laughing gas” – is now a class C drug in the UK under the misuse of drugs act 1971. Possession will be illegal by the end of 2023. If, However, you can get hold of some (or have some to get rid of) a recent paper by Cornella group has you covered.1
Sustainability and repurposing are buzzwords increasingly finding their way into synthetic organic chemistry papers. Inert gases with high global warming potentials are close to the top of the list in terms of “problems looking for solutions”, however, by definition, these materials are difficult to “fix” into a usable reactive form. Nitrous oxide- a potential oxygen transfer reagent- falls into this category. High temperatures and pressures (>150°C, 50-200 bar) are required for its activation (it works nicely as an oxidant in rocket engines). These conditions are perhaps manageable in the petrochemical industry but are not compatible with the complex (sensitive) molecules we make and process every day in the pharma and fine chemical industry. N2O has a global warming potential >300 times that of CO2 and a half-life of over 100 years in the atmosphere. It surprised me to learn that the effect of nitrous oxide on stratospheric ozone is comparable with the now largely banned CFC’s. This is no laughing matter. Around 10% of global production of N2O is as a by-product from adipic acid production.2b However most of the world production of waste N2O comes from agriculture, and not surprisingly the amount being generated is increasing with increasing global demand for food.2Nitrification and denitrification of microorganisms is an important pathway for N2O production. This 2-step sequence involves nitrification via aerobic conversion of ammonia to nitrate (NO3–) and denitrification via conversion of nitrates to N2O and N2 under anoxic conditions.2c Nitrous oxide deoxygenation, particularly electrochemical reduction using metals, is an active area of research.2dAny process that addresses the environmental burden of gas accumulation is a step forward.
Nitrous oxide is an interesting reagent for organic synthesis, with examples of its use both as an oxygen donor and, to a lesser degree, a nitrogen source. An example of the later is production of sodium azide by reaction of sodium amide with N2O- a process I reviewed in an earlier post.3 Synthetic chemistry with nitrous oxide has been reviewed in Chem. Soc. Rev. by Kay Severin- a key player in this area.4 It is a weak sigma-donor and pi-acceptor so metal ligation and activation for homogeneous catalysis is not easy. That said, the Cornella group have developed a mild, room-temperature catalytic Ni-C insertion reaction for making valuable and synthetically useful phenols from aryl halides.1
The early proof of concept for the work came from a paper published in 1987 by Hillhouse.5 He showed that, unlike traditional metal-oxo reactivity, treating a hafnium hydride complex with N2O resulted in insertion of oxygen in the Hf-Ph bond generating Hf-O-Ph with extrusion of nitrogen. The problem in this case was a competing process in which oxygen was transferred to a metal bound hydride generating the Hf-OH complex, the later being the dominant pathway (Figure 1). Mechanistically, an organometallic Baeyer–Villiger (OMBV)-type process is in operation, whereby the anionic carbon migrates to the coordinated oxygen atom.
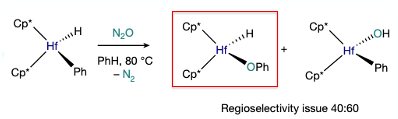
Cornella’s paper describes leveraging this mechanistic paradigm for oxygen transfer from nitrous oxide to an aryl halide generating a phenol. Unlike traditional methods that use water and base in a standard oxidative addition/reductive elimination process, the metal in the OMBV-type reaction remains at the same oxidation state and requires an exogenous reducing agent to reduce the metal and generate the phenol (Figure 2).
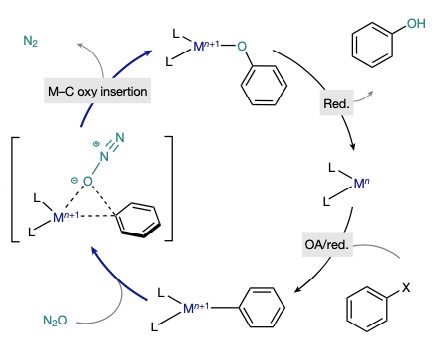
The metal selected to underpin the process was Nickel- a suitable system for shuttling between oxidation states via single electron transfer. Zinc /sodium iodide was demonstrated to be a suitable reducing agent for closing the catalytic cycle. Selective C-O bond formation was achieved using a tridentate pyridine ligand with NiBr2 (glyme) (Figure 3). The ligand was prepared in 82% yield by reaction of 6-bromo-2,2’-dipyridine with pyrazole using a copper (I)catalyst (0.1 eqv. Cu2O, 2 eqv. Cs2CO3, DMSO, 100°C, 16 hrs). In the presence of the metal complex, N2O acted as an electrophlic oxygen source and smooth conversion of an aryl halide to phenol was realised under mild conditions (25°C, 1-2 atm), particularly and uniquely with base-sensitive substrates, including a hydrolytically vulnerable ester and a base-sensitive sulfone (Figure 4). A few drug-like intermediates are also part of the example set, including a derivative of the SGLT-2 inhibitor Empagliflozin (used to treat type-2 diabetes) containg a doubly benzylic carbon atom (Figure 4). Another impressive example is the conversion of a derivativ of the anti-cholesterol drug Ezetimibe to a phenol in the presence of a chiral secondary alcohol, and ester, and an acid/base sensitive 4-membered lactam ring.
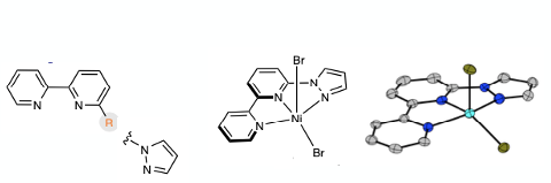
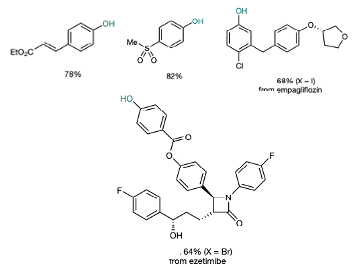
The conditions described in the paper are robust and notably work well with heavily functionalized substrates. The process is mechanistically distinct in that the phenol products are generated via an electrophilic oxygen source rather than the traditional nucleophilic OH- coupling partners. The consequence is that base sensitive substrates survive under neutral reaction conditions.
Finally, if you want to see something spectacular-all fire and brimstone- check out the “barking dog” demonstration. Reaction of N2O with carbon disulfide. I first saw this reaction demonstrated by a kamikaze teacher at secondary school and its one of those experiments that piqued my interest in chemistry. The rest, as they say, is history.6
See you next time.
References:
- Catalytic synthesis of phenols with nitrous oxide: J. Cornella et al, Nature 2022, 604, 677-683; Highlighted by H. M. Huang et al, Nat. Chem. 2022, 14, 846-848
- a) Global agriculture and nitrous oxide emissions: D. Reay et al, Nature Clim. Change 2012, 2, 410–416; b) A comprehensive qualification of global nitrous oxide sources and sinks: Tian et al, Nature 2020, 586, 248-256; b) A critical review on the progress and challenges to a more sustainable, cost competitive synthesis of adipic acid: J. Rios et al, Green Chem. 2021, 23, 3172-3190; c) The nitrogen cycle: processes, players and human impact: A. Bernhard, Nature Education 2010, 3, 25; d) Electrochemical reduction of N2O with a molecular copper catalyst: J. Martinez et al, ACS Catal. 2023, 13, 12673-12680
- Sodium azide- sonic boom boy: J. Studley blog March 2023 https://www.scientificupdate.com/process-chemistry-articles/sodium-azide-sonic-boom-boy/
- a) Synthetic chemistry with nitrous oxide: K. Severin, Soc. Rev. 2015, 44, 6375-6386; b) Nitrous oxide as a diazo transfer reagent: the synthesis of triazolopyridines: K. Severin et al, Chem. Commun. 2021, 57, 11537-11540; c) Homogeneous catalysis with nitrous oxide: K. Severin, Trends Chem. 2023, 5, 574-576; d) Modern chemistry of nitrous oxide: A. V. Leont’ev et al, Russ. Chem. Rev. 2001, 70,91-104; e) Homogeneous catalysis with nitrous oxide: K. Severin, Trends in chemistry 2023, 5, 574-576
- Selective O-transfer from nitrous oxide to hydride and aryl ligands of Bis(pentamethylcyclopentadienyl) hafnium derivatives: G. L. Hillhouse et al, J. Am. Chem. Soc. 1987, 109, 5538-5539
- University of Nottingham, periodic table of videos: https://www.youtube.com/watch?v=c3fJRRCAIdk