Deoxyfluorination remains amongst the most frequently used method for preparing alkyl fluoro compounds.1 The reaction typically involves activation of a leaving group followed by SN2 (but occasionally SN1) reaction with fluoride ion. This is usually accompanied with significant elimination side-reactions and can frequently be low yielding. The original deoxyfluorination reagents such as DAST (diethylaminosulfur-trifluoride) and Dexofluor (Bis(2-methoxyethyl)aminosulfur trifluoride) are toxic and difficult to handle, particularly at scale, leading to the development of flow chemistry processes and use of microreactors.2 That said, these reagents have had a significant impact on the access to alkyl fluoro compounds, with a notable increase in fluorine-containing clinical candidates and drugs appearing in the 1970’s and 80’s once these reagents gained traction in the synthetic community.3 DAST was reported by chemists at Dupont in 1975 as a bench stable alternative to SF4.4
Improvements in handleability and safety with the introduction of PhenoFluor, AlkylFluor and reagents such as PyFluor and XtalFluor have partially addressed some of these issues,5 however their remains considerable scope for improvement- particularly transposition of tertiary alcohol substrates.
Mechanistically this should be achievable by switching pathways. Formation of a radical at a tertiary carbon should be facile and the radical intermediate a good nucleophile to trap with an electrophilic fluorine source. This gives an orthogonal approach to the reaction of an activated leaving group with a poorly nucleophilic fluoride ion. Generation of a radical intermediate of this type is perfectly suited for visible light photoredox, leveraging low-temperature activation and mild reaction conditions.
Not Surprisingly the MacMillan group have just published a very nice paper on photoredox-catalyzed deoxyfluorination of activated alcohols with everybody’s favourite fluorine source- the commercially available, inexpensive, stable reagent Selectfluor (Tetrahedron 2019, 75, 4222-4227).6 Generation of the alkyl radical from the corresponding alcohol was achieved by conversion to the oxalate half-ester and double decarboxylation- methodology developed through a collaboration between the MacMillan and Overman groups (Scheme 1).7

A proposed mechanism is outlined in Scheme 2. Upon exposure to visible light the heteroleptic (mixed ligand) complex (Ir[df(CF3)ppy]2(dtbbpy)PF6) forms a long-lived excited state species. Reduction of selectfluor (to initiate the catalytic cycle) generates an Ir(IV) oxidant via a single electron transfer (SET) process. Reaction of this species with the activated alcohol results in loss of CO2, generation of an alkyl radical and return of the catalyst to its ground state.
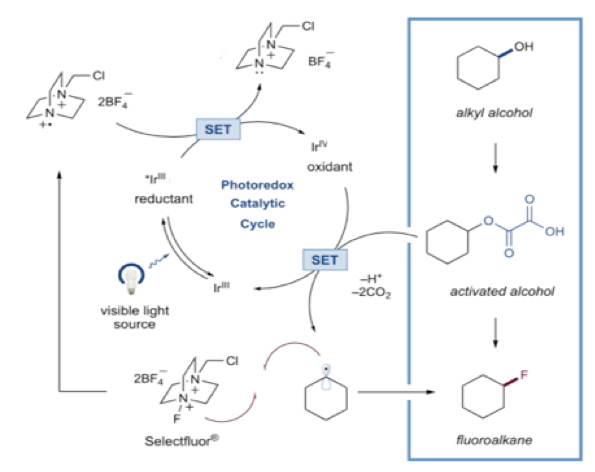
Fluorine atom transfer to the alkyl radical gives the fluoroalkane product and a radical cation that re-oxidizes the triplet excited iridium catalyst to the Ir(IV) species, cycling the process. 8 Photochemical quenching experiments using the Stern-Volmer procedure showed that selectfluor is able to oxidise the excited state Ir(III) species Ir(Iv) adding weight to the proposed mechanism.
Optimization (utilizing the oxalate derived from 2-decanol) revealed the best reaction conditions were using 1mol% of photocatalyst (Ir[dF(OMe)ppy]2(dtbbpy)PF6) , Na2HPO4 as base in acetone/water with a 34W blue LED lamp (Scheme 3).9 A range of secondary and tertiary alcohols could be converted to their corresponding fluorides in good yield (67-80% isolated yield). Protected amines, esters and amides were stable to the reaction conditions, however for tertiary alcohols higher yields were observed with a lower power carbon filament lamp and less Selectfluor reagent.
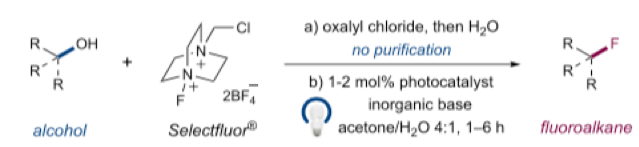
Deoxyfluorination of a range of natural product alcohols and sterically hindered bridge structures, including steroids, proceeded in good yields with good selectivity.
Its good to see a new twist on the deoxyfluorination reaction, complimentary to traditional nucleophilic SN2 processes, providing access to tertiary alkyl fluorides under mild conditions with a reagent that most people are familiar with.
Organofluorine Chemistry: Synthesis, Applications, Sources and Sustainability
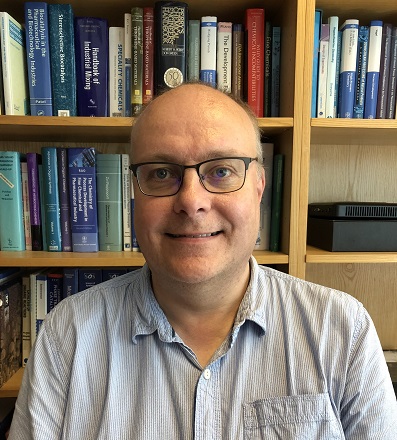
Organofluorine compounds are frequently encountered in the pharmaceutical, agrochemical and fine chemical industries. Often the selective introduction of a fluorine atom or functional group into a molecule can be challenging, particularly at scale, with chemists often designing synthetic routes in which fluorine is introduced through strategic sourcing of raw materials rather than synthetically.
Scientific Update offer a new two-day course that aims to provide an overview of fluorination technology and synthetic methods- both traditional and state of the art- highlighting industrial application. Detailed case studies are used to provide context and workshops enable delegates to familiarize themselves with the chemistry described. For more information contact me at [email protected]
References:
- Recent Developments in the Deoxyfluorination of Alcohols and Phenols: New Reagents, Mechanistic Insights, and Applications: W. Hu et al, Synthesis 2017, 49, 4917.
- The use of diethylaminosulfur-trifluoride (DAST) for fluorination in a continuous flow microreactor: S. Ley et al, Synlett 2008, 14, 2111-2114; Aminosulfurtrifluorides: relative thermal stability: W. Middleton et al, J. Fluorine Chem. 1989, 42, 137-143
- Archived Webinar, J. Studley, Scientific Update, April 2019 https://www.scientificupdate.com/webinar_events/fluorine-synthetic-methods-for-selective-introduction-into-small-molecule-drugs/20190424/
- Good partnership between sulfur and fluorine: sulfur-based fluorination and fluoroalkylation reagents for organic synthesis: J. Hu et al, Chem. Rev. 2015, 115, 765-825.
- Late-stage deoxyfluorination of alcohols with PhenoFluor: T. Titter et al, J. Am. Chem. Soc. 2013, 135, 2470-2473; AlkylFluor: deoxyfluorination of alcohols: N. Goldberg et al, Org. Lett. 2016, 18, 6102-6104; PyFluor: A low-cost, stable, and selective deoxyfluorination reagent: A. Doyle et al, J. Am. Chem. Soc.2015, 137, 9571-9574; XtalFluor-E: A useful and versatile reagent in organic transformations: M. Heravi et al, J. Fluorine Chem. 2019, 225, 11-20.
- Selectfluor reagent F-TEDA-BF4 in action: tamed fluorine at your service R. Banks, J. Fluorine Chem. 1998, 87, 1-17; Recent advances in the application of Selectfluor F-TEDA-BF4as a versatile mediator or catalyst in organic synthesis: S. Stavber Molecules 2011, 16, 6432-6464.
- Oxalates as Activating Groups for Alcohols in Visible Light Photoredox Catalysis: Formation of Quaternary Centers by Redox-Neutral Fragment Coupling: MacMillan and Overmann et al, J. Am. Chem. Soc. 2015, 137, 11270-11273.
- Fluorine Transfer to Alkyl Radicals: G. Sammis et al, J. Am. Chem. Soc. 2012, 134, 4026-4029.
- The oxalate half ester was prepared using oxalyl chloride then water and was used without further purification.
- Lisa M. JarvisC&EN 2019, 97 https://cen.acs.org/pharmaceuticals/drug-development/new-drugs-2018/97/i3. Fluorine-containing small molecules: Vitrakvi, Lorbrena, Xofluza, Talzenna, Vizimpro, Pifeltro, Xerava, Orlissa, Krintafel, Tibsovo, Tpoxx, Mektovi, Akynzeo, Tavalisse, Erleada, Symdeko and Biktarvy.